Discovery of Muon neutrino
The very first experiment using a beam of high-energy neutrinos originated in one of the daily coffee breaks at the Pupin laboratory, where faculty and research students would relax together for half an hour. In this stimulating atmosphere around Nobel Prizewinners T.D. Lee, C.N. Yang (Nobel prize for physics 1957) and others at the end of the 1950s, the need to find a feasible method of studying the effect of weak forces at high energies was discussed. Hitherto it had only been possible to study processes of radioactive decay, spontaneous processes at necessarily relatively low energies. Beams of all common particles (electrons, protons and neutrons) were discussed. While these are relatively simple to produce, they were found to be unusable for this purpose. The apparently hopeless situation suddenly changed when Melvin Schwartz proposed that it ought to be possible to produce and use a beam of neutrinos. During the next two years he, together with Leon Lederman and Jack Steinberger, worked on the proposal in order to achieve a sufficiently intense beam of neutrinos free from all other types of particle, and to design a detector for measuring neutrino reactions.
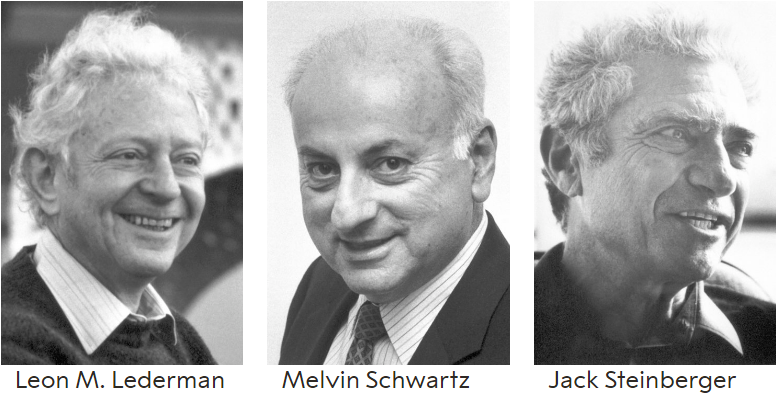
The neutrinos in the Columbia experiment were produced in the decay in the flight of charged pi-mesons. In a first step protons were accelerated to high velocities and directed at a target of the metal beryllium. As the next step high-velocity pi-mesons were produced in a forward-directed beam. Mesons are radioactive, and they decayed into a muon and a neutrino each when allowed to travel a path of free flight, which was set at 21 metres. In this step high-energy neutrinos were produced as a forward-directed beam, still containing quantities of leftover pi-mesons and myons which had been formed at the same time. To eliminate these unwanted particles completely from the beam, a 13.5-metre-thick wall of steel was needed. The material came from scrapped warships. The measuring device (detector) was built behind the wall, which of course was transparent to the neutrinos. So that the detector should not be entirely transparent, it was thought best to build it as a 10-ton spark chamber, then a new and fairly untested type. The detector consisted of a large number of aluminium plates with spark gaps between them. A muon or an electron produced by a neutrino in one of the aluminium plates photographed its own track as a series of sparks, using a special self-exposing device.
In order to understand how the weak force works and to explore the possible existence of two neutrinos, it was necessary to study neutrino interactions at high energies. Leon Lederman, Melvin Schwartz and Jack Steinberger were working to achieve a sufficiently intense neutrino beam for several years. This neutrino beam was produced in several steps:
- Protons were accelerated to an energy of 15 GeV (1 GeV = 109 eV) in the Brookhaven accelerator AGS (Alternating Gradient Synchrotron) on Long Island, USA.
- The intense proton beam was directed onto a target of beryllium. In each collision a handful of particles were produced, mainly pi-mesons.
- The many pi-meson decays resulted in a collimated beam of muons and neutrinos.
- The neutrinos, the muons and the surviving pi-mesons crashed into a 13 m thick steel shield, which stopped all particles except the neutrinos.
The steel shield, which was made of armour plates from scrapped warships, was essential for the experiment. All particles, except the neutrinos, had to be prevented from reaching the detector, as they could give rise to a large number of particle reactions and completely swamp the incredibly few neutrino reactions. The pi-mesons, which interact through the strong force, were stopped after less than half a metre of armour plates, while the muons, which interact mainly through the electromagnetic force, penetrated much further. The steel shield, which had a weight of about 2000 tons, was dimensioned to stop the penetrating muons.
For the neutrinos to have a fair chance to interact, i.e. collide, the detector had to be massive. Furthermore, the charged particles produced in the collision had to be detected. Leon Lederman, Melvin Schwartz and Jack Steinberger studied different types of detectors before they decided, in 1960, to construct a 10 ton spark chamber. The detector was divided into 90 plates of aluminium, each an inch thick. The spaces between the plates were filled with neon gas.
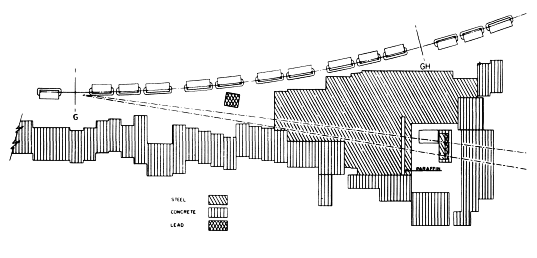
113 events were observed, excluding those originated outside the chambers, those which were not originated within a fiducial volume and those of single tracks where the extrapolation of the track backwards for two gaps did not remain within the fiducial volume or where the production angle relative to the neutrino line of flight was more than 60°. These events were classified as follows:
- 49 short single tracks: If interpreted as muons, they are single tracks whose visible momentum is less then 300 MeV/c. They won’t be considered acceptable events, because they include energetic muons that leave the chamber, low-energy neutrino events and the bulk of the neutron produced background.
- 34 single muons of more than 300 MeV/c.
- 22 vertex events whose origin is characterized by more than one track. In all of these events an energy release is observable.
- 8 showers. They are not acceptable events, because they are too irregular to be µ mesons.
Only the 56 events of the second and third case above were finally considered.
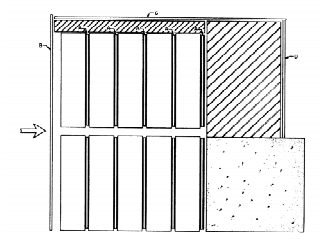
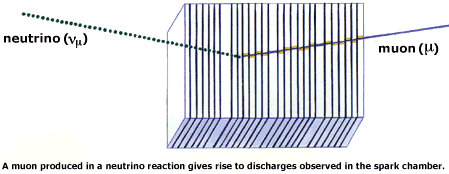
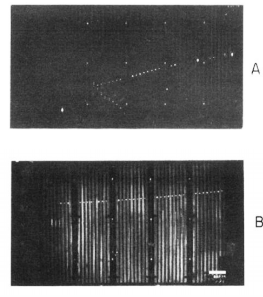
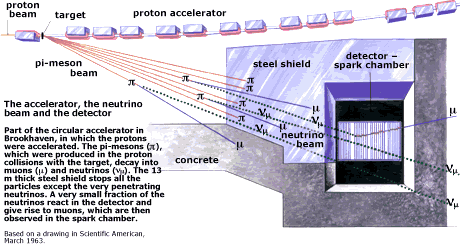
As neutrino interactions are very rare, the background from other processes was disturbing, particularly the background caused by the penetrating muons from the cosmic radiation. The background was reduced by keeping the experiment active only during the short pulses, 3 microseconds, when the accelerator delivered particles. During the 8 month experiment period they accumulated data for 25 days, but because of the short pulses, the efficient time for the detector was only 6 seconds.
Each neutrino pulse contained 107 neutrinos. During the experiment a total of 1014 neutrinos went through the detector during the six seconds it was active. When a reaction took place, a high voltage was applied to the spark chamber and a discharge occurred where the produced charged particles had passed. The sparks were photographed and gave a picture of the particles’ path through the detector. A few neutrino interactions were registered with a muon observed in the spark chamber. These results showed that the neutrinos, produced together with muons, give rise to muons, not electrons.
A burning problem had arisen at the time of the experiment regarding the measurements of muon radioactive decay. The measurement results, to which Jack Steinberger and Bruno Pontecorvo among others contributed, disagreed with accepted theoretical calculations. The problem was addressed by many researchers, among them G. Feinberg and T.D. Lee, as well as methodologically by Pontecorvo, and they indicated that one way out of the dilemma would be the existence of two entirely different types of neutrino.
If the neutrinos in the Columbia experiment beam were identical to the neutrinos common in beta decay, the reactions in the detector should convert the neutrino to a fast electron as often as to a fast muon. On the other hand only muons would result if there were two different kinds of neutrino. The prizewinners and their collaborators arranged their detector so that the cause of the spark tracks could be interpreted. The results showed that only muons were produced by the neutrinos in the beam, no electrons. Thus there exists a new type of neutrino that forms an intimate pair with the muon. Consequently the electron forms its own delimited family with its neutrino.
The discovery thus had immediate consequences. Knowledge of the role of the family concept and the great importance of the method within elementary particle physics has grown during the time that has elapsed since the discovery was made. A question that is still current is whether or not small departures from strict family membership occur.
The experiment of the Nobel Prize winners not only showed that the muon and the muon neutrino form a pair, it also showed that the other two leptons, the electron and the electron neutrino, form a pair. The grouping in pairs of elementary particles is fundamental in particle physics and became even more significant after the establishment of the so called standard model.
Several experiments have since been carried out both in the USA and Europe in order to study the innermost structure of matter and to further explore the weak force.
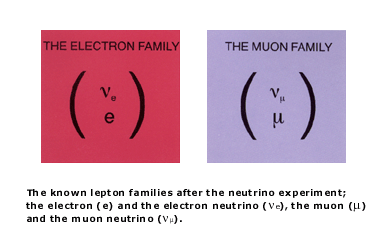


REFERENCES
Brookhaven National Laboratory. Available in: https://www.bnl.gov/bnlweb/history/nobel/1988.php. Access in: 17/11/2018.
CERN. Available in: https://indico.cern.ch/event/402462/attachments/806290/1104933/JournalClub.pdf. Access in: 17/11/2018.
Nobel Prize. Available in: https://www.nobelprize.org/prizes/physics/1988/9557-the-hunt-for-the-muon-neutrino/. Access in: 17/11/2018.
Università degli Studi di Udine. Available in: http://www.fisica.uniud.it/~cobal/Site/muon_neutrino.pdf. Access in: 17/11/2018.
0 comments
Sign in or create a free account