Electroweak interaction
Electroweak theory, in physics, the theory that describes both the electromagnetic force and the weak force. Superficially, these forces appear quite different. The weak force acts only across distances smaller than the atomic nucleus, while the electromagnetic force can extend for great distances (as observed in the light of stars reaching across entire galaxies), weakening only with the square of the distance. Moreover, comparison of the strength of these two fundamental interactions between two protons, for instance, reveals that the weak force is some 10 million times weaker than the electromagnetic force. Yet one of the major discoveries of the 20th century has been that these two forces are different facets of a single, more-fundamental electroweak force.
The electroweak theory arose principally out of attempts to produce a self-consistent gauge theory for the weak force, in analogy with quantum electrodynamics (QED), the successful modern theory of the electromagnetic force developed during the 1940s. There are two basic requirements for the gauge theory of the weak force. First, it should exhibit an underlying mathematical symmetry, called gauge invariance, such that the effects of the force are the same at different points in space and time. Second, the theory should be renormalizable; i.e., it should not contain nonphysical infinite quantities.
During the 1960s Sheldon Lee Glashow, Abdus Salam, and Steven Weinberg independently discovered that they could construct a gauge-invariant theory of the weak force, provided that they also included the electromagnetic force.
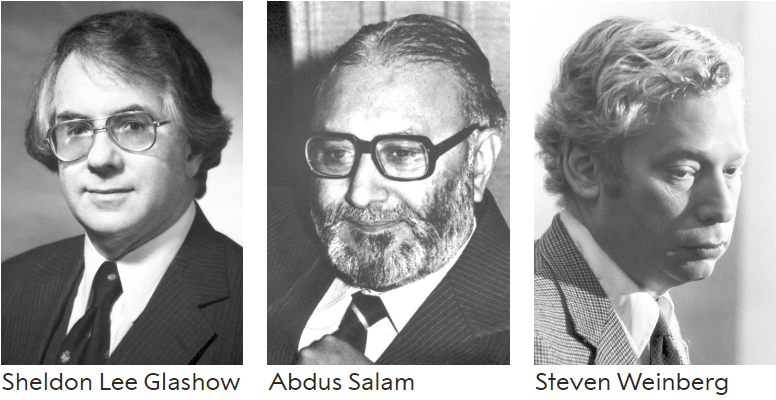
Their theory required the existence of four massless “messenger” or carrier particles, two electrically charged and two neutral, to mediate the unified electroweak interaction. The short range of the weak force indicates, however, that it is carried by massive particles. This implies that the underlying symmetry of the theory is hidden, or “broken,” by some mechanism that gives mass to the particles exchanged in weak interactions but not to the photons exchanged in electromagnetic interactions. The assumed mechanism involves an additional interaction with an otherwise unseen field, called the Higgs field, that pervades all space.
The discovery of the radioactivity of certain heavy elements towards the end of last century, and the ensuing development of the physics of the atomic nucleus, led to the introduction of two new forces or interactions: the strong and the weak nuclear forces. Unlike gravitation and electromagnetism these forces act only at very short distances, of the order of nuclear diameters or less. While the strong interaction keeps protons and neutrons together in the nucleus, the weak interaction causes the so-called radioactive beta-decay. The typical process is the decay of the neutron: the neutron, with charge zero, is transformed into a positively charged proton, with the emission of a negatively charged electron and a neutral, massless particle, the neutrino.
Although the weak interaction is much weaker than both the strong and the electromagnetic interactions, it is of great importance in many connections. The actual strength of the weak interaction is also of significance. The energy of the sun, all-important for life on earth, is produced when hydrogen fuses or burns into helium in a chain of nuclear reactions occurring in the interior of the sun. The first reaction in this chain, the transformation of hydrogen into heavy hydrogen (deuterium), is caused by the weak force. Without this force solar energy production would not be possible. Again, had the weak force been much stronger, the life span of the sun would have been too short for life to have had time to evolve on any planet. The weak interaction finds practical application in the radioactive elements used in medicine and technology, which are in general beta-radioactive, and in the beta-decay of a carbon isotope into nitrogen, which is the basis for the carbon-14 method for dating of organic archaeological remains.
Theories of weak interaction
A first theory or weak interaction was put forward already in 1934 by the Italian physicist Fermi. However, a satisfactory description of the weak interaction between particles at low energy could be given only after the discovery in 1956 that the weak force differs from the other forces in not being reflection symmetric; in other words, the weak force makes a distinction between left and right. Although this theory was valid only for low energies and thus had a restricted domain of validity, it suggested a certain kinship between the week and the electromagnetic interactions.
In a series of separate works in the 1960’s Glashow, Salam and Weinberg developed a theory which is applicable also at higher energies, and which at the same time unifies the weak and electromagnetic interactions in a common formalism.
Glashow, Salam and Weinberg started from earlier contributions by other scientists. Of special importance was a generalization of the so-called gauge principle for the description of the electromagnetic interaction. This generalization was worked out around the middle of the 1950’s by Yang and Mills in USA. After the fundamental work in the 1960’s the theory has been further developed. An important contribution was made in 1971 by the young Dutch physicist van’t Hooft.
The theory predicts among other things the existence of a new type of weak interaction, in which the reacting particles do not change their charges. This behaviour is similar to what happens in the electromagnetic interaction, and one says that the interaction proceeds via a neutral current. One should contrast this with the beta-decay of the neutron, where the charge is altered when the neutron is changed into a proton.
First observation of the weak neutral current
The first observation of an effect of the new type of weak interaction was made in 1973 at the European nuclear research laboratory, CERN, in Geneva in an experiment where nuclei were bombarded with a beam of neutrinos. Since then a series of neutrino experiments at CERN and at the Fermi Laboratory near Chicago have given results in good agreement with theory. Other laboratories have also made successful tests of effects of the weak neutral current interaction. Of special interest is a result, published in the summer of 1978, of an experiment at the electron accelerator at SLAC in Stanford, USA. In this experiment the scattering of high energy electrons on deuterium nuclei was studied and an effect due to a direct interplay between the electronmagnetic and weak parts of the unified interaction could be observed.
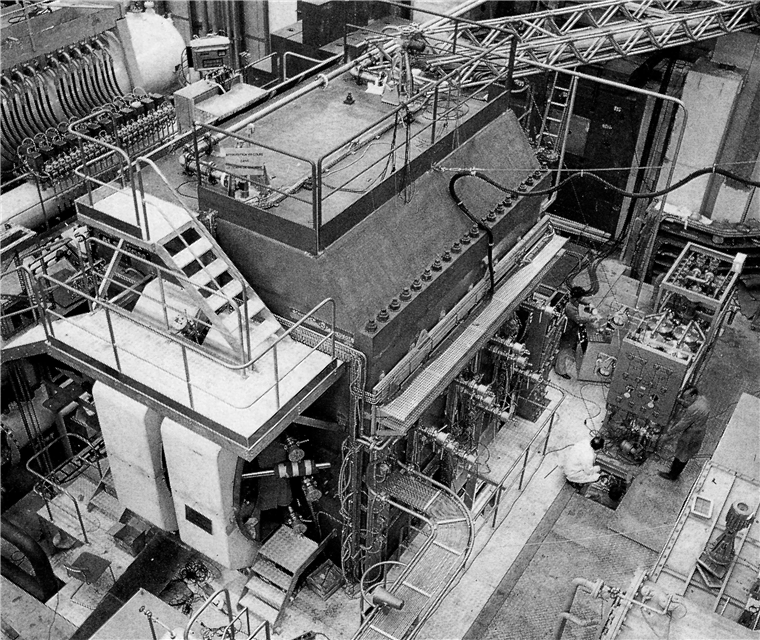
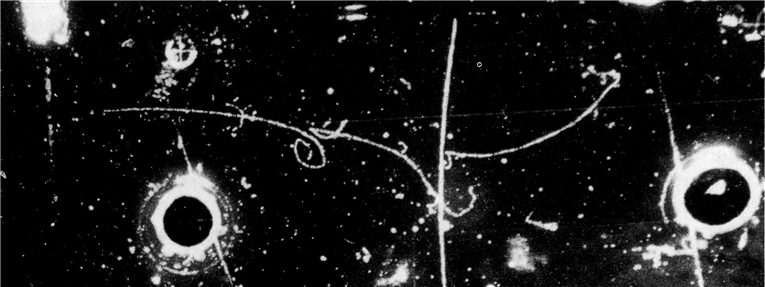
Interaction carried by particles
An important consequence of the theory is that the weak interaction is carried by particles having some properties in common – with the photon, which carries the electromagnetic interaction between charged particles. These so-called weak vector bosons differ from the massless photon primarily by having a large mass; this corresponds to the short range of the weak interaction. The theory predicts masses of the order of one hundred proton masses, but today’s particle accelerators are not powerful enough to be able to produce these particles.
In the early 1970s Gerardus ’t Hooft and Martinus Veltman provided the mathematical foundation to renormalize the unified electroweak theory proposed earlier by Glashow, Salam, and Weinberg. Renormalization removed the physical inconsistencies inherent in earlier calculations of the properties of the carrier particles, permitted precise calculations of their masses, and led to more-general acceptance of the electroweak theory. The existence of the force carriers, the neutral Z particles and the charged W particles, was verified experimentally in 1983 in high-energy proton-antiproton collisions at the European Organization for Nuclear Research (CERN). The masses of the particles were consistent with their predicted values.
Formulation
Mathematically, the unification is accomplished under an SU(2) × U(1) gauge group. The corresponding gauge bosons are the three W bosons of weak isospin from SU(2) (W1, W2, and W3), and the B boson of weak hypercharge from U(1), respectively, all of which are massless.
In the Standard Model, the W± and Z0 bosons, and the photon, are produced by the spontaneous symmetry breaking of the electroweak symmetry from SU(2) × U(1)Y to U(1)em, caused by the Higgs mechanism (see also Higgs boson). U(1)Y and U(1)em are different copies of U(1); the generator of U(1)em is given by Q = Y/2 + T3, where Y is the generator of U(1)Y (called the weak hypercharge), and T3 is one of the SU(2) generators (a component of weak isospin).
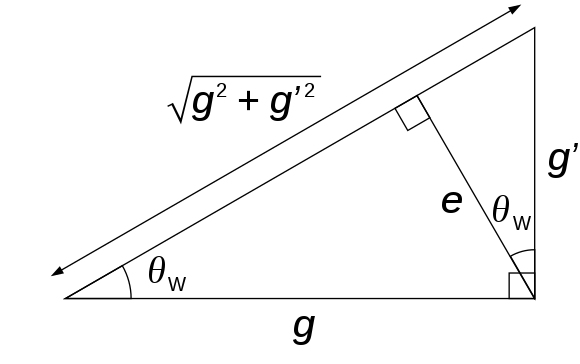
The spontaneous symmetry breaking makes the W3 and B bosons coalesce into two different bosons – the Z0 boson, and the photon (γ),

where θW is the weak mixing angle. The axes representing the particles have essentially just been rotated, in the (W3, B) plane, by the angle θW. This also introduces a mismatch between the mass of the Z0 and the mass of the W± particles (denoted as MZ and MW, respectively),
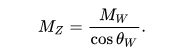
The W1 and W2 bosons, in turn, combine to give massive charged bosons
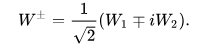
The distinction between electromagnetism and the weak force arises because there is a (nontrivial) linear combination of Y and T3 that vanishes for the Higgs boson (it is an eigenstate of both Y and T3, so the coefficients may be taken as −T3 and Y): U(1)em is defined to be the group generated by this linear combination, and is unbroken because it does not interact with the Higgs.
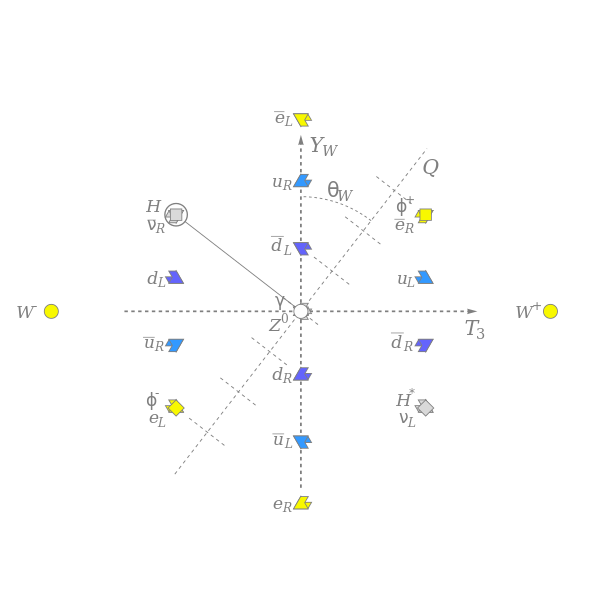
The characteristics of the unified electroweak force, including the strength of the interactions and the properties of the carrier particles, are summarized in the Standard Model of particle physics.
Weinberg, Salam and Glashow on physics - CERN JournalA MODEL OF LEPTONS - Steven Weinberger PaperWeak and Electromagnetic Interactions - ABDUS SALAMThe Electroweak Force, Grand Unification and Superunification - Abdus SalamTHE RENORMALIZABILITY OF VECTOR MESON INTERACTIONS - SHELDON L. GLASHOWGenesis of Electroweak Unification - Lecture.pdf



REFERENCES
Encyclopædia Britannica. Available in: https://www.britannica.com/science/electroweak-theory. Access in: 11/11/2018.
Nobel Prize. Available in: https://www.nobelprize.org/prizes/physics/1979/summary/. Access in: 11/11/2018.
CERN Document Server. Available in: http://cds.cern.ch/record/1730597/files/vol20-issue8-p350-e.pdf. Access in: 11/11/2018.
Wikipedia. Available in: https://en.wikipedia.org/wiki/Electroweak_interaction#cite_ref-2. Access in: 11/11/2018.
0 comments
Sign in or create a free account