Nuclear Fission
Nuclear fission of heavy elements was discovered on December 17, 1938 by German Otto Hahn and his assistant Fritz Strassmann, and explained theoretically in January 1939 by Lise Meitner and her nephew Otto Robert Frisch.
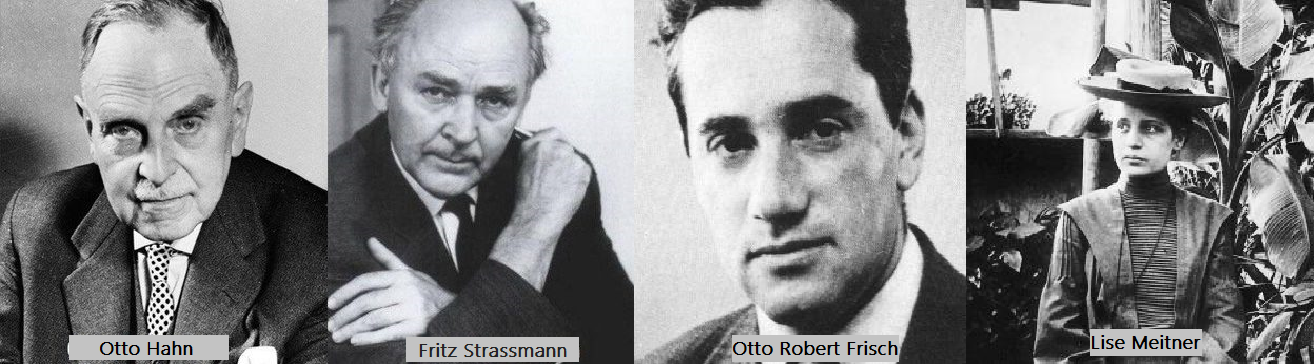
Frisch named the process by analogy with biological fission of living cells. For heavy nuclides, it is an exothermic reaction which can release large amounts of energy both as electromagnetic radiation and as kinetic energy of the fragments (heating the bulk material where fission takes place). In order for fission to produce energy, the total binding energy of the resulting elements must be more negative (greater binding energy) than that of the starting element.
Fission is a form of nuclear transmutation because the resulting fragments are not the same element as the original atom. The two nuclei produced are most often of comparable but slightly different sizes, typically with a mass ratio of products of about 3 to 2, for common fissileisotopes. Most fissions are binary fissions (producing two charged fragments), but occasionally (2 to 4 times per 1000 events), three positively charged fragments are produced, in a ternary fission. The smallest of these fragments in ternary processes ranges in size from a proton to an argon nucleus.
Apart from fission induced by a neutron, harnessed and exploited by humans, a natural form of spontaneous radioactive decay (not requiring a neutron) is also referred to as fission, and occurs especially in very high-mass-number isotopes. Spontaneous fission was discovered in 1940 by Flyorov, Petrzhak and Kurchatov in Moscow, when they decided to confirm that, without bombardment by neutrons, the fission rate of uranium was indeed negligible, as predicted by Niels Bohr; it was not.
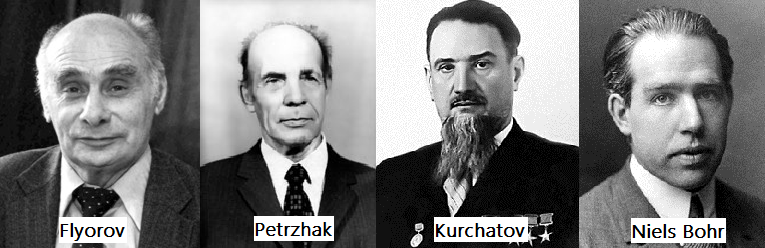
The unpredictable composition of the products (which vary in a broad probabilistic and somewhat chaotic manner) distinguishes fission from purely quantum-tunneling processes such as proton emission, alpha decay, and cluster decay, which give the same products each time. Nuclear fission produces energy for nuclear power and drives the explosion of nuclear weapons. Both uses are possible because certain substances called nuclear fuels undergo fission when struck by fission neutrons, and in turn emit neutrons when they break apart. This makes a self-sustaining nuclear chain reaction possible, releasing energy at a controlled rate in a nuclear reactor or at a very rapid, uncontrolled rate in a nuclear weapon.
In engineered nuclear devices, essentially all nuclear fission occurs as a "nuclear reaction" — a bombardment-driven process that results from the collision of two subatomic particles. In nuclear reactions, a subatomic particle collides with an atomic nucleus and causes changes to it. Nuclear reactions are thus driven by the mechanics of bombardment, not by the relatively constant exponential decay and half-life characteristic of spontaneous radioactive processes.
Many types of nuclear reactions are currently known. Nuclear fission differs importantly from other types of nuclear reactions, in that it can be amplified and sometimes controlled via a nuclear chain reaction (one type of general chain reaction). In such a reaction, free neutrons released by each fission event can trigger yet more events, which in turn release more neutrons and cause more fissions.
The chemical element isotopes that can sustain a fission chain reaction are called nuclear fuels, and are said to be fissile. The most common nuclear fuels are 235U (the isotope of uranium with an atomic mass of 235 and of use in nuclear reactors) and 239Pu (the isotope of plutonium with an atomic mass of 239). These fuels break apart into a bimodal range of chemical elements with atomic masses centering near 95 and 135 u (fission products). Most nuclear fuels undergo spontaneous fission only very slowly, decaying instead mainly via an alpha-beta decay chain over periods of millennia to eons. In a nuclear reactor or nuclear weapon, the overwhelming majority of fission events are induced by bombardment with another particle, a neutron, which is itself produced by prior fission events.
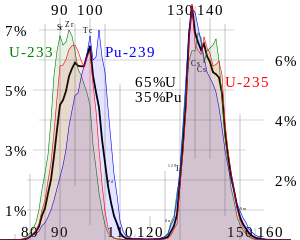
Nuclear fissions in fissile fuels are the result of the nuclear excitation energy produced when a fissile nucleus captures a neutron. This energy, resulting from the neutron capture, is a result of the attractive nuclear force acting between the neutron and nucleus. It is enough to deform the nucleus into a double-lobed "drop," to the point that nuclear fragments exceed the distances at which the nuclear force can hold two groups of charged nucleons together and, when this happens, the two fragments complete their separation and then are driven further apart by their mutually repulsive charges, in a process which becomes irreversible with greater and greater distance. A similar process occurs in fissionable isotopes (such as uranium-238), but in order to fission, these isotopes require additional energy provided by fast neutrons (such as those produced by nuclear fusion in thermonuclear weapons).
When an incident subatomic projectile is absorbed by a heavy nucleus, the resulting compound nucleus is produced in a very excited state. The excitation energy E* of the compound nucleus equals the binding energy of the incident particle to it plus the kinetic energy of the incident projectile, less a negligible recoil energy of the compound nucleus. The excited compound nucleus is very unstable, with a lifetime of about 10-14 s. The "nuclear fluid" of such an excited nucleus undergoes large oscillations and deformations in shape. If the compound nucleus is sufficiently excited, it may, during one of its oscillations in shape, deform into an elongated or dumbbell configuration in which the two ends Coulombically repel each other and the nuclear forces, being very short ranged, are no longer able to hold the two ends logether. The two ends then separate (scission) within ~ 10-20 s into two nuclear pieces, repelling each other with such tremendous Coulombic force that many of the orbital electrons are left behind. Two highly charged fission fragments are thus created.
For example, in neutron-induced fission of 235U, the reaction sequence, immediately following scission of the compound nucleus, is
n + 235U92 → 236U*92 → YH+n + YL+m + (n + m)e-
where the YH and YL indicate, respectively, the heavy and light primary fission products, and the ionic charge n and m on the fission fragments is about 20. U* is unstable.
The primary fission fragments are produced in such highly excited nuclear states that neutrons can "boil" off them. Anywhere from 0 to about 8 neutrons, denoted by vp, evaporate from the primary fission fragments within about 10-17 s of the scission or splitting apart of the compound nucleus. These neutrons are called prompt fission neutrons, hence the subscript p in vp, in order to distinguish them from delayed neutrons emitted at later times during radioactive decay of the fission products.
Following prompt neutron emission, the fission fragments are still in excited states, but with excitation energies insufficient to cause particle emission. They promptly decay to lower energy levels only by the emission of prompt gamma rays γp. This emission is usually completed within about 2x10-14 s after the emission of the prompt neutrons.
The highly charged fission fragments pass through the surrounding medium causing millions of Coulombic ionization and excitation interacts with the electrons of the medium. As the fission fragments slow, they gradually acquire electrons reducing their ionization charge, until, by the time they are stopped, they have become electrically neutral atoms. This transfer of the fission fragments' kinetic energy to the ambient medium takes about 10-12 s. Thus, after about 10-12 s following scission, the fission reaction may be written
n + 235U92 → 236U*92 → YH + YL + vp(1n0) + γp
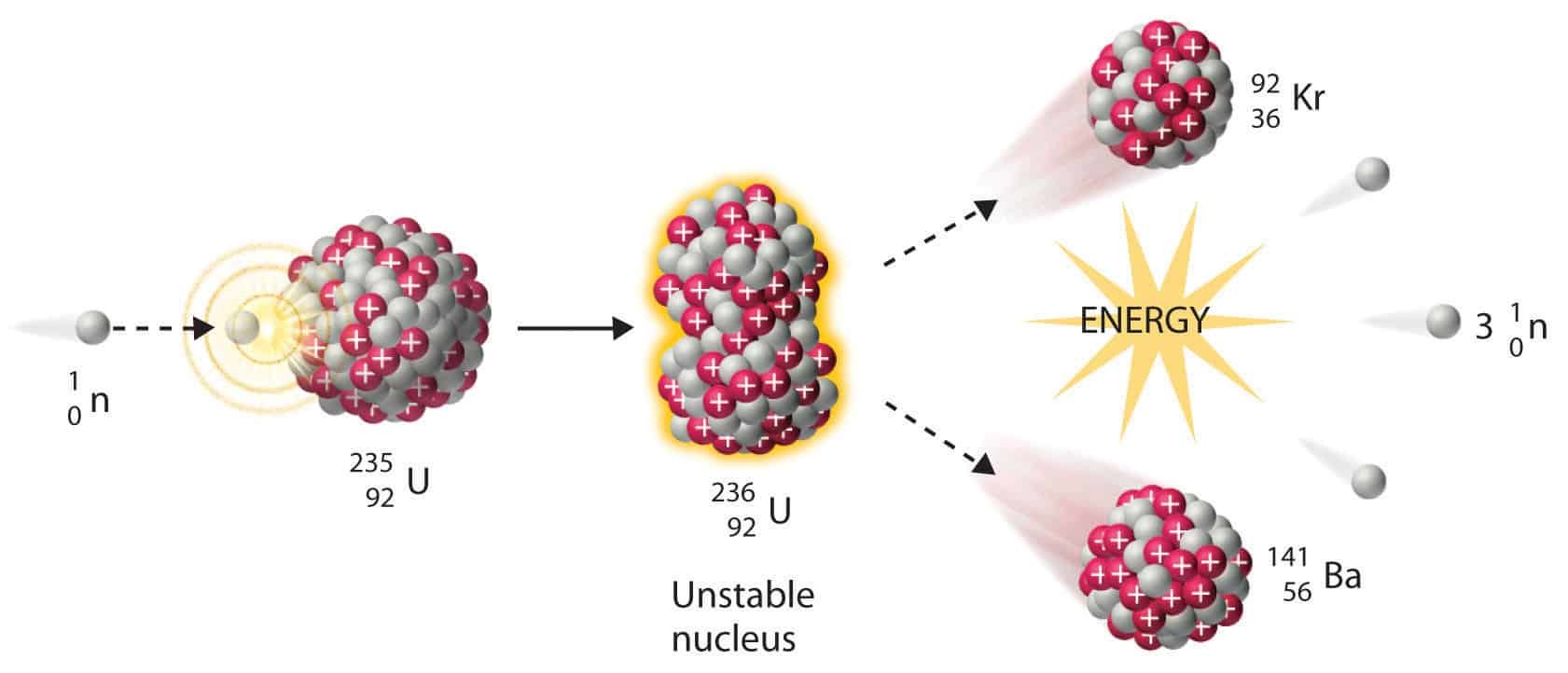
Induced fission reaction. A neutronis absorbed by a uranium-235 nucleus, turning it briefly into an excited uranium-236 nucleus, with the excitation energy provided by the kinetic energy of the neutron plus the forces that bind the neutron. The uranium-236, in turn, splits into fast-moving lighter elements (fission products) and releases a small amount of free neutrons. At the same time, one or more "prompt gamma rays" are produced, as well.
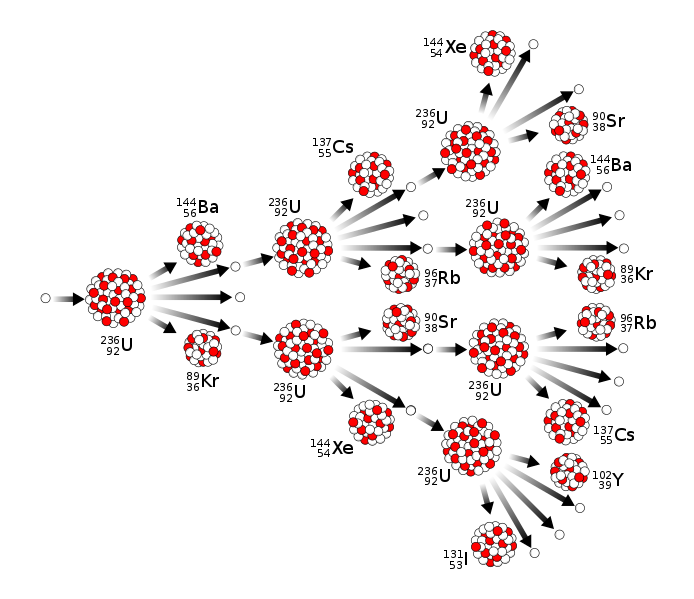
After prompt neutron and gamma ray emission, the fission fragments are termed fission products. The fission products are still radioactively unstable since they still have too many neutrons compared to protons to form stable atoms. They thus form the start of decay chains whose members radioactively decay, usually by isobaric β- decay, until a stable end-nuclide is reached. The half-lives of the fission product daughters range from fractions of a second to many thousands of years. The proper management of these long-lived fission products is a major challenge to proponents of nuclear fission power.
Fission Reactors
Critical fission reactors are the most common type of nuclear reactor. In a critical fission reactor, neutrons produced by fission of fuel atoms are used to induce yet more fissions, to sustain a controllable amount of energy release. Devices that produce engineered but non-self-sustaining fission reactions are subcritical fission reactors. Such devices use radioactive decay or particle accelerators to trigger fissions.
Critical fission reactors are built for three primary purposes, which typically involve different engineering trade-offs to take advantage of either the heat or the neutrons produced by the fission chain reaction:
- power reactors are intended to produce heat for nuclear power, either as part of a generating station or a local power system such as a nuclear submarine.
- research reactors are intended to produce neutrons and/or activate radioactive sources for scientific, medical, engineering, or other research purposes.
- breeder reactors are intended to produce nuclear fuels in bulk from more abundant isotopes. The better known fast breeder reactormakes 239Pu (a nuclear fuel) from the naturally very abundant 238U (not a nuclear fuel). Thermal breeder reactors previously tested using 232Th to breed the fissile isotope 233U (thorium fuel cycle) continue to be studied and developed.
While, in principle, all fission reactors can act in all three capacities, in practice the tasks lead to conflicting engineering goals and most reactors have been built with only one of the above tasks in mind. (There are several early counter-examples, such as the Hanford N reactor, now decommissioned). Power reactors generally convert the kinetic energy of fission products into heat, which is used to heat a working fluid and drive a heat engine that generates mechanical or electrical power. The working fluid is usually water with a steam turbine, but some designs use other materials such as gaseous helium. Research reactors produce neutrons that are used in various ways, with the heat of fission being treated as an unavoidable waste product. Breeder reactors are a specialized form of research reactor, with the caveat that the sample being irradiated is usually the fuel itself, a mixture of 238U and 235U. For a more detailed description of the physics and operating principles of critical fission reactors, see nuclear reactor physics. For a description of their social, political, and environmental aspects, see nuclear power.
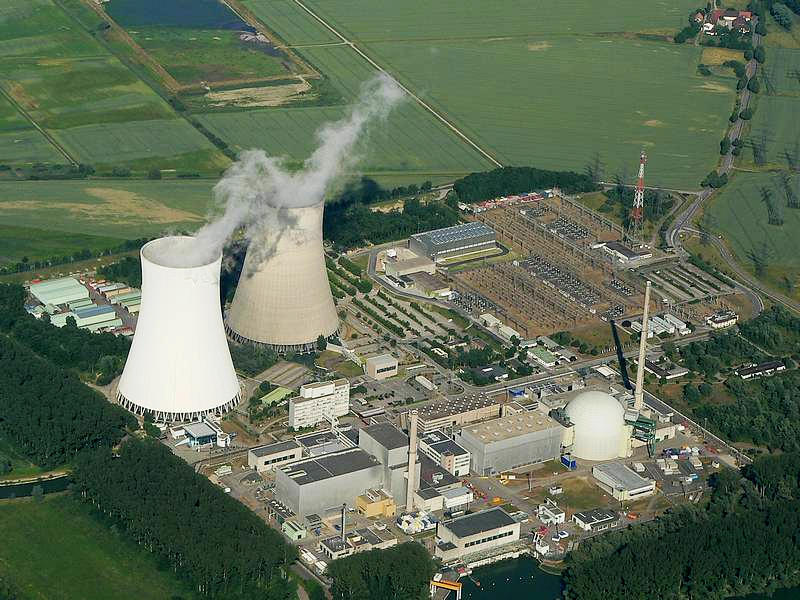




REFERENCES
SHULTIS, J. Kenneth; FAW, Richard E. FUNDAMENTALS OF NUCLEAR SCIENCE AND ENGINEERING. Marcel Dekker, Inc., New York, 2002.p. 150-153.
Wikipedia. Available in: https://en.wikipedia.org/wiki/Nuclear_fission. Access in: 01/10/2018.
0 comments
Sign in or create a free account