Fullerene 60
Fullerene, also called buckminsterfullerene, any of a series of hollow carbon molecules that form either a closed cage (“buckyballs”) or a cylinder (carbon “nanotubes”). The first fullerene was discovered in 1985 by Sir Harold W. Kroto (one of the authors of this article) of the United Kingdom and by Richard E. Smalley and Robert F. Curl, Jr., of the United States.
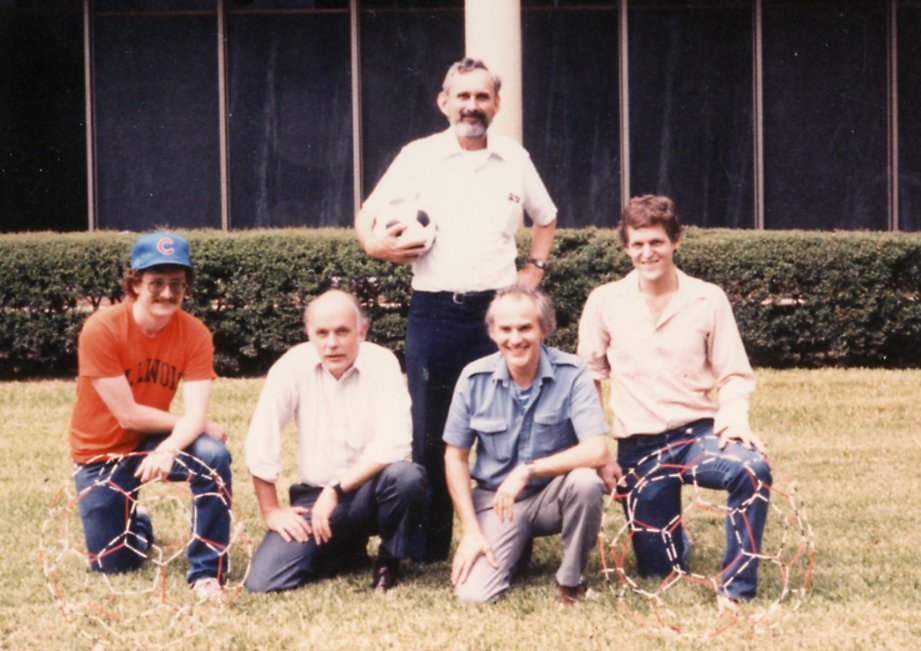
Using a laser to vaporize graphite rods in an atmosphere of helium gas, these chemists and their assistants obtained cagelike molecules composed of 60 carbon atoms (C60) joined together by single and double bonds to form a hollow sphere with 12 pentagonal and 20 hexagonal faces—a design that resembles a football, or soccer ball. In 1996 the trio was awarded the Nobel Prize for their pioneering efforts. The C60 molecule was named buckminsterfullerene (or, more simply, the buckyball) after the American architect R. Buckminster Fuller, whose geodesic dome is constructed on the same structural principles. The elongated cousins of buckyballs, carbon nanotubes, were identified in 1991 by Iijima Sumio of Japan.
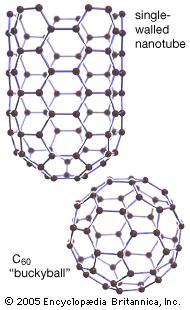
The fullerenes, particularly the highly symmetrical C60 sphere, have a beauty and elegance that excites the imagination of scientists and nonscientists alike, as they bridge aesthetic gaps between the sciences, architecture, mathematics, engineering, and the visual arts. Prior to their discovery, only two well-defined allotropes of carbon were known—diamond (composed of a three-dimensional crystalline array of carbon atoms) and graphite (composed of stacked sheets of two-dimensional hexagonal arrays of carbon atoms). The fullerenes constitute a third form, and it is remarkable that their existence evaded discovery until almost the end of the 20th century. Their discovery has led to an entirely new understanding of the behaviour of sheet materials, and it has opened an entirely new chapter of nanoscience and nanotechnology—the “new chemistry” of complex systems at the atomic scale that exhibit advanced materials behaviour. Nanotubes in particular exhibit a wide range of novel mechanical and electronic properties. They are excellent conductors of heat and electricity, and they possess an astonishing tensile strength. Such properties hold the promise of exciting applications in electronics, structural materials, and medicine. Practical applications, however, will only be realized when accurate structural control has been achieved over the synthesis of these new materials.
Buckminsterfullerenes
During the period 1985–90 Kroto, working with colleagues at the University of Sussex, Brighton, England, used laboratory microwave spectroscopy techniques to analyze the spectra of carbon chains. These measurements later led to the detection, by radioastronomy, of chainlike molecules consisting of 5 to 11 carbon atoms in interstellar gas clouds and in the atmospheres of carbon-rich red giant stars. On a visit to Rice University, Houston, Texas, in 1984, Curl, an authority on microwave and infrared spectroscopy, suggested that Kroto see an ingenious laser–supersonic cluster beam apparatus developed by Smalley. The apparatus could vaporize any material into a plasma of atoms and then be used to study the resulting clusters (aggregates of tens to many tens of atoms). During the visit, Kroto realized that the technique might be used to simulate the chemical conditions in the atmosphere of carbon stars and so provide compelling evidence for his conjecture that the chains originated in stars. In a now-famous 11-day series of experiments conducted in September 1985 at Rice University by Kroto, Smalley, and Curl and their student coworkers James Heath, Yuan Liu, and Sean O’Brien, Smalley’s apparatus was used to simulate the chemistry in the atmosphere of giant stars by turning the vaporization laser onto graphite. The study not only confirmed that carbon chains were produced but also showed, serendipitously, that a hitherto unknown carbon species containing 60 atoms formed spontaneously in relatively high abundance. Attempts to explain the remarkable stability of the C60 cluster led the scientists to the conclusion that the cluster must be a spheroidal closed cage in the form of a truncated icosahedron—a polygon with 60 vertices and 32 faces, 12 of which are pentagons and 20 hexagons. They chose the imaginative name buckminsterfullerene for the cluster in honour of the designer-inventor of the geodesic domes whose ideas had influenced their structure conjecture.
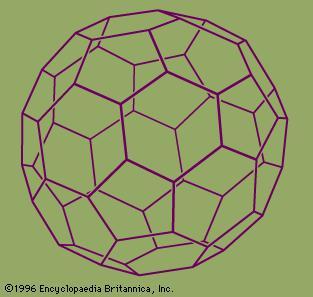
From 1985 to 1990, a series of studies indicated that C60, and also C70, were indeed exceptionally stable and provided convincing evidence for the cage structure proposal. In addition, evidence was obtained for the existence of other smaller metastable species, such as C28, C36, and C50, and experimental evidence was provided for “endohedral” complexes, in which an atom was trapped inside the cage. Experiments showed that the size of an encapsulated atom determined the size of the smallest surrounding possible cage. In 1990 physicists Donald R. Huffman of the United States and Wolfgang Krätschmer of Germany announced a simple technique for producing macroscopic quantities of fullerenes, using an electric arc between two graphite rods in a helium atmosphere to vaporize carbon. The resulting condensed vapours, when dissolved in organic solvents, yielded crystals of C60. With fullerenes now available in workable amounts, research on these species expanded to a remarkable degree, and the field of fullerene chemistry was born.
The C60 molecule undergoes a wide range of novel chemical reactions. It readily accepts and donates electrons, a behaviour that suggests possible applications in batteries and advanced electronic devices. The molecule readily adds atoms of hydrogen and of the halogen elements. The halogen atoms can be replaced by other groups, such as phenyl (a ring-shaped hydrocarbon with the formula C6H5 that is derived from benzene), thus opening useful routes to a wide range of novel fullerene derivatives. Some of these derivatives exhibit advanced materials behaviour. Particularly important are crystalline compounds of C60 with alkali metals and alkaline earth metals; these compounds are the only molecular systems to exhibit superconductivity at relatively high temperatures above 19 K. Superconductivity is observed in the range 19 to 40 K, equivalent to −254 to −233 °C or −425 to −387 °F.
Particularly interesting in fullerene chemistry are the so-called endohedral species, in which a metal atom (given the generic designation M) is physically trapped inside a fullerene cage. The resulting compounds (assigned the formulas M@C60) have been extensively studied. Alkali metals and alkaline earth metals as well as early lanthanoids may be trapped by vaporizing graphite disks or rods impregnated with the selected metal. Helium (He) can also be trapped by heating C60 in helium vapour under pressure. Minute samples of He@C60 with unusual isotope ratios have been found at some geologic sites, and samples also found in meteorites may yield information on the origin of the bodies in which they were found.
Potential Applications Of Fullerenes
The discovery of C60 has led to a paradigm shift in the understanding of graphite, in particular graphene sheets on a small scale. It is now known that the most stable form of a carbon aggregate, containing tens to several thousands of atoms, is a closed buckyball or nanotube. This new understanding is not restricted to pure carbon but also applies to other sheet-forming materials such as boron nitride, which can also form nanotubes. Closed fullerene structures, incorporating sulfides of such metals as tungsten and molybdenum, exhibit excellent solid-lubricant properties. Conducting carbon nanotubes may be coated with sheaths of metal sulfides to produce tiny insulated electrical wire.
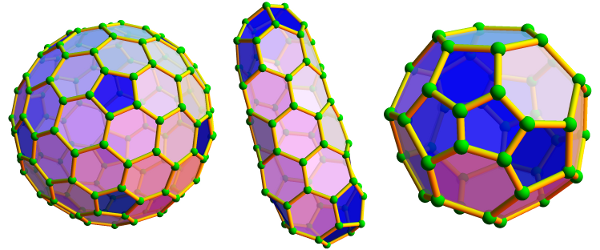
Fullerenes and nanotubes have engendered much excitement, especially with regard to possible future applications, but so far such applications have been few and far between. Nanotubes in particular may well bring about a revolution in materials science. For example, if SWNTs can be made in bundles of 100 billion, then a material will be produced that may approach the limits of tensile strength possible for any known material involving the chemical bond. In practice, no material approaches its theoretical “intrinsic strength,” because of breakdowns brought on by the propagation of microscopic defects through the material. A bundle of nanotubes, however, may bypass this problem, as microscopic defects may anneal along the length of a particular tube and certainly should not propagate across the bundle—thus avoiding the problems that occur in conventional materials. Estimates of potential tensile strength vary, but it is predicted that a 1-metre rod may reach 50 to 100 times the strength of steel at one-sixth the weight. The impact of such a material on civil engineering, building construction, aircraft, and automobiles would be spectacular. In order to realize this potential, however, new processes will have to be discovered that can produce long (more than 1 metre), perfectly ordered bundles in which all 100 billion nanotubes preferably have the same diameter and atomic arrangement. At present the technology to achieve this does not exist; indeed, it is not even obvious what strategy might be used to reach this goal. More realistically, carbon-nanotube composite materials exhibiting improved behaviour over standard carbon-fibre composites are likely in the near term. In addition, applications on a small scale should be feasible for medical purposes—for instance, the strength of individual nanotubes may prove useful in microsurgery or nanosurgery.
Naming
The discoverers of the Buckminsterfullerene (C60) allotrope of carbon named it after Richard Buckminster Fuller, a noted architectural modeler who popularized the geodesic dome. Since buckminsterfullerenes have a similar shape to those of such domes, they thought the name appropriate.
As the discovery of the fullerene family came after buckminsterfullerene, the shortened name 'fullerene' is used to refer to the family of fullerenes. The suffix "-ene" indicates that each C atom is covalently bonded to three others (instead of the maximum of four), a situation that classically would correspond to the existence of bonds involving two pairs of electrons ("double bonds").
Types of fullerene
Since the discovery of fullerenes in 1985, structural variations on fullerenes have evolved well beyond the individual clusters themselves. Examples include:
- Buckyball clusters: smallest member is C (unsaturated version of dodecahedrane) and the most common is C;
- Nanotubes: hollow tubes of very small dimensions, having single or multiple walls; potential applications in electronics industry
- Megatubes: larger in diameter than nanotubes and prepared with walls of different thickness; potentially used for the transport of a variety of molecules of different sizes;
- polymers: chain, two-dimensional and three-dimensional polymers are formed under high-pressure high-temperature conditions; single-strand polymers are formed using the Atom Transfer Radical Addition Polymerization (ATRAP) route.
- nano"onions": spherical particles based on multiple carbon layers surrounding a buckyball core proposed for lubricants;
- linked "ball-and-chain" dimers: two buckyballs linked by a carbon chain;
- fullerene rings.
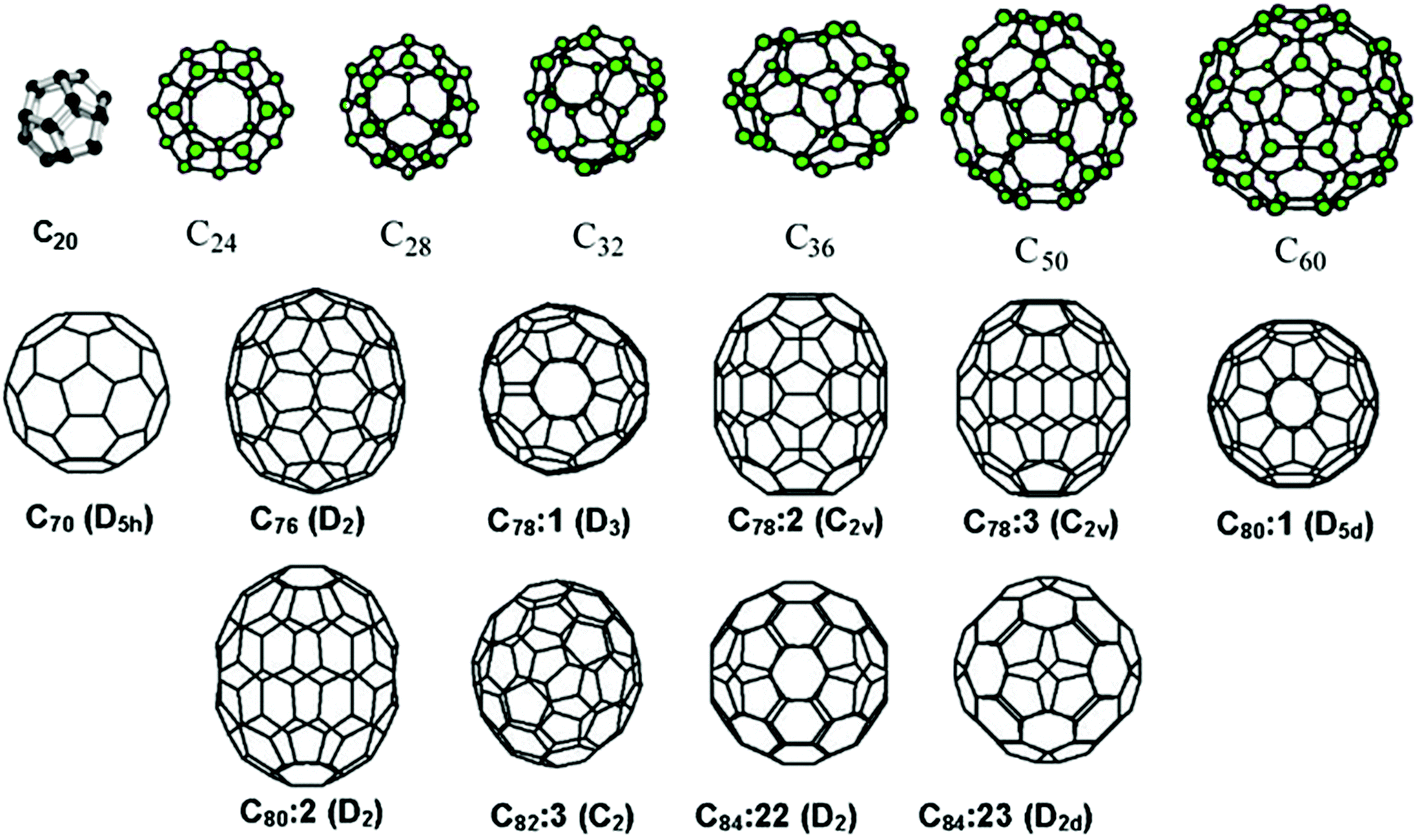



REFERENCES
Encyclopædia Britannica. Available in: https://www.britannica.com/science/fullerene. Access in: 28/10/2018.
Wikiédia. Available in: https://en.wikipedia.org/wiki/Fullerene. Access in: 28/10/2018.
SATTLER, Klaus D. Handbook of Nanophysics: Clusters and Fullerenes. Chapter 23.
0 comments
Sign in or create a free account